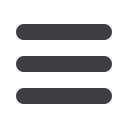
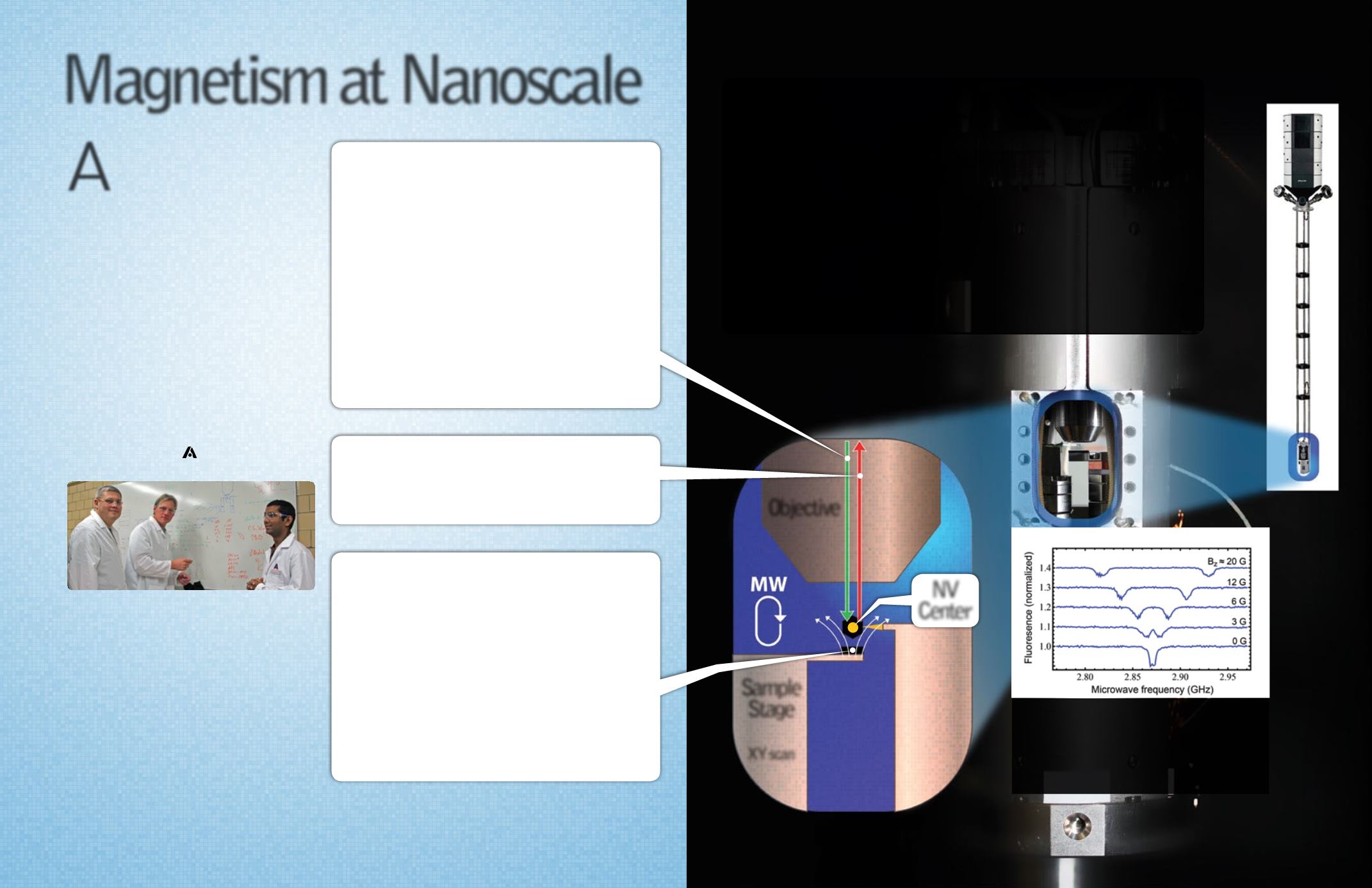
10
Inquir y I s sue 1
|
201 5
Inqui r y I s sue 1
|
2015
11
NV Centers
Usually, diamonds are most valued when they’re perfect and big. But physicists see
special value in diamonds’ tiny flaws: a certain kind of imperfection, called a nitrogen-
vacancy (NV) center, serves as a very sensitive sensor of the magnetic field exactly at the
location of the NV center. NV centers are created when a carbon atom is substituted with
a nitrogen atom. When there is a missing atom or a “vacancy” nearby the nitrogen atom,
this forms the stable pair called the nitrogen-vacancy center.
What makes NV centers so useful? Physicists know a lot about how NV centers work.
(In fact, Ames Laboratory is home to one of the world’s leading experts on NV centers,
theoretical physicist Viatcheslav Dobrovitski.) Scientists know how much energy it takes
to push electrons from the lowest energy, or ground state, to an excited state and, more
importantly, how much energy will be released in form of a red photon when the electron
relaxes back to the low-energy level. NV centers’ well-defined quantum energy levels are
extremely sensitive to a magnetic field. This sensitivity enables the NV-magnetoscope
to detect very small magnetic fields – such as that produced by nano- and mesoscale
magnetic materials, for example – by reading optical fluorescence emitted by the excited
NV centers.
Objective
s the demand grows for ever smaller,
smarter electronics, so does the demand for
understanding materials’ behavior at ever-
smaller scales. Ames Laboratory physicists
are building a unique optical magnetometer to probe
magnetism at the nano- and mesoscale.
The device, called a NV-magnetoscope, makes
use of the unique quantum mechanical properties of
nitrogen-vacancy (NV) centers in diamond. The low-
temperature NV-magnetoscope setup incorporates
a confocal microscope (CFM) and an atomic-force
scanning microscope (AFM).
The NV-magnetoscope will be able to sense the
extremely weak magnetic fields of just a handful
of electrons with the spatial resolution of about 10
nanometers.
“We want to determine magnetic textures more
precisely than ever before, at smaller scales than ever
before,” saidAmes Laboratory physicist Ruslan Prozorov.
“Our hope is to understand nano- and mesoscale
magnetism, learn how to control it and, eventually, use
that to create a new generation of technologies.”
Experimental physicists Ruslan Prozorov (left)
and Naufer Nusran (right) and theoretical physicist
Viatcheslav Dobrovitski (center) are collaborating
to bring the preciseness of quantum mechanics to
measuring nanoscale magnetism in Ames Laboratory’s
NV-magnetoscope. The development of the NV-
magnetoscope is a flagship effort of Ames Laboratory’s
“Magnetic Nanosystems: Making, Measuring,
Modeling and Manipulation” research team, led by
Prozorov. Theoretical work is done by Dobrovitski and
hands-on experimental work is done by a dedicated
postdoctoral researcher, Nusran, who has built
the experimental setup, the first NV-centers optical
magnetometer fitted into the low-temperature AFM/CFM
system, first of its kind in the United States acquired from
Germany’s Attocube.
A
Sample
Stage
XYscan
Green Laser Light Excites the NV Center
“Electrons start at low-energy quantum states. And the green
laser light ‘kicks’ them to a high excited state. The rules of quantum
mechanics say that those electrons must return back to the lower
energy level. If an electron was excited from a non-magnetic level,
it always emits red light. However, if it was excited from one of
the low-energy magnetic levels, it most likely relaxes back without
any emission.
Microwave radiation is used to scramble electrons between
low-energy magnetic and non-magnetic states, reaching maximum
population of the magnetic states when the interlevel energy
difference matches microwave energy. Therefore, by scanning
microwave frequency, red fluorescence will cause double-dip
spectra, corresponding to two magnetic energy levels, split by the
magnetic field (called Zeeman splitting). The distance between the
dips is proportional to the magnetic field at the location of an NV
center,” said Prozorov.
“NV-magnetoscope measures the intensity
of red light fluorescence as a function of
microwave radiation frequency. The distance
between the two depressions in the plot tells us
about the strength of the magnetic field in the
location of the NV center,” said Prozorov.
Detector Counts Red Photons
As excited electrons lose energy and return back to the low-
energy state, they emit red light. A detector counts the number of
red photons.
NV Centers “Feel” Sample’s Magnetic Fields
A roughly 100-nanometer-long diamond containing NV centers
is attached to the AFM tip. The confocal microscope focuses on a
single NV center, collecting red photons only from one tiny area while
blocking out outside “noise.” The sample of interest is scanned below
the NV center. The NV center “feels” the variation of magnetic fields
produced by the sample.
“When the sample of interest is brought close enough to an NV
center, the sample’s magnetic field is extended to the location of
the NV center and affects the center’s quantum energy levels. By
accurately moving the sample in two dimensions close to the NV
center, we can reconstruct the magnetic field intensity map produced
by the sample. This, in turn, gives access to the magnetic properties
of the sample itself,” said Prozorov.
Magnetism
atNanoscale
NV
Center