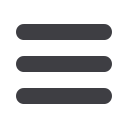
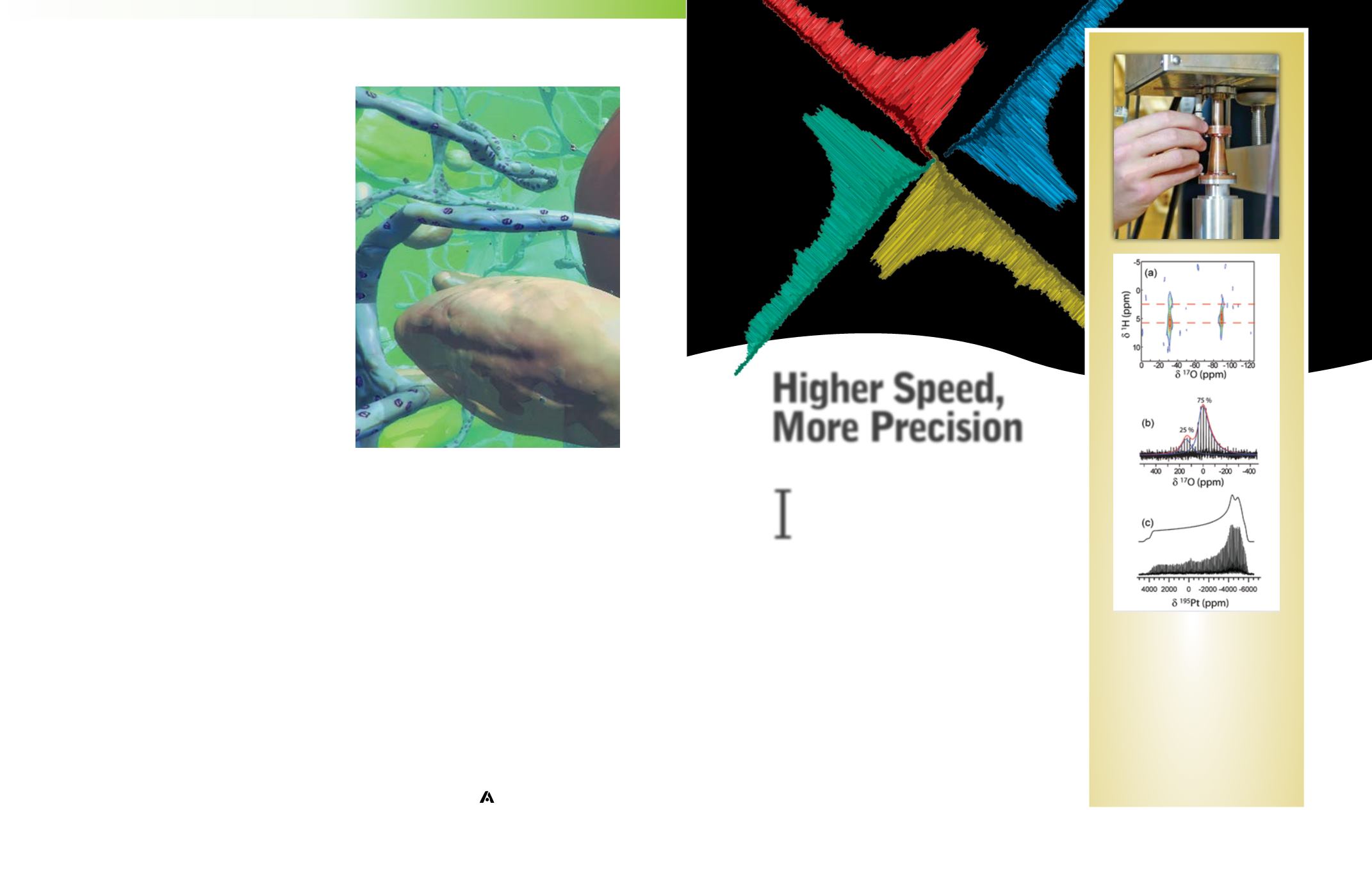
12
Inqui r y I ssue 2
|
2015
Inqui r y I s sue
2
| 201 5
13
material,” said Emily Smith, Ames Laboratory scientist and
ISU associate professor of chemistry. “That means using
imaging techniques that provide exquisite spatial resolution
that shows you structures and molecules. You also want to
get chemical information, to know exactly what chemical
compounds are present. “
“The STED gives us good spatial resolution so we can
look at subcellular structures,” Smith continued. “The
Raman imaging gives us the chemical analysis so we know,
for example, what fatty acids are present.”
In addition to these techniques by Smith’s group and
ISU researcher Jacob Petrich, the team is aided by ISU
associate professor of chemistry Art Winter who has
developed molecular probes that selectively “light up” or
“turn on” certain elements within the cell that can then be
captured by the STED imaging. Ames Laboratory scientists
Sam Houk and Young-Jin Lee provide additional chemical
analysis through advanced mass-spectroscopy methods, and
provide a means of validating the images obtained by the
other imaging technologies.
“One of the downsides to using the techniques we’ve
developed is that you can get so much information that one
person couldn’t begin to sort out what’s important,” Smith said.
“That’s where the computational techniques developed by Eve
Syrkin Wurtele and Diane Bassham come into play to sort out
the needle of useful information in the haystack of data to
address the particular biological question we’re posing.”
Wurtele and Bassham, both professors of Genetics,
Development and Cell Biology at ISU, use an integrated
experimental/biocomputational approach to understand the
factors that regulate plant development and composition.
That includes an award-winning computer visualization
program – yep, a video game – calledMeta!Blast. Data can be
imported into the program and it provides a visual depiction
of what’s taking place within the plant at the appropriate
cellular or molecular scale.
“The idea is that you have this visual environment
that’s new to most people and you can do things like scan
structures or molecules if you don’t understand them,
get an explanation of the analytical technologies, and
see multiple vantage points,” Wurtele said. “The various
chemical and biological (analytical) techniques let you
create an image of the plant and its molecular components
that is at a resolution from a multicellular level, like the
surface of a leaf, to the cellular, into the organelles within
individual cells, and ultimately the individual molecules.”
All involved say the project perfectly melds the strengths
of ISU and Ames Laboratory.
“In our group, we’re all experts in our own domains,
but I don’t think there’s one person who fully understands
the other people’s technologies,” Wurtele said. “The idea
behind this is to create a system where the actual data that’s
obtained at different resolutions and spatial compartments
is integrated so that anyone is able to access the data in an
understandable form.”
“It’s a highly collaborative project,” Nikolau added, “and a
very good fit between the biological and the analytical sides.”
“We developed these various analytical techniques for
other purposes,” Smith concluded. “With this project,
we’re able to apply them to biological materials. ISU is
extremely strong in plant science and Ames Lab has vast
analytical expertise, and it’s been great to see this synergy
develop. Hopefully we can leverage that down the road on
future projects.”
This image from the Meta!Blast program shows structures
within the cells of plant. The program is used to visually
represent huge volumes of data collected from living plants to
accurately depict what takes place chemically and physically
within the plant.
All involved say the project
perfectly melds the strengths
of ISU andAmes Laboratory.
n just a little over a year of operation,
Ames
Laboratory’s dynamic nuclear polarization (DNP) solid-
state nuclear magnetic resonance (NMR) spectrometer
has successfully characterized materials at the atomic
scale level with more speed and precision than ever possible
before. Spectra for materials important to catalysis, solar
energy, and hydrogen storage have helped scientists better
understand how these materials work.
Conventional NMR detects the responses of atomic
nuclei to direct excitation by radio frequency waves. DNP-
NMR offers faster and more detailed spectra over traditional
NMR by first exciting unpaired electrons at their microwave
resonance frequency and then transferring the resulting spin
polarization to the material’s nuclei. This additional step
results in much stronger signal intensities from these “hyper-
polarized” nuclei than is available by conventional means.
The advanced capabilities of DNP-NMR have already
made it possible for Ames Laboratory NMR experts to
answer questions that were out of reach before.
For example, in a paper in the Journal of the American
Chemical Society, Ames Laboratory scientist Marek
Pruski and his research team reported on DNP studies
of natural abundance
17
O in several materials. Oxygen is
practically undetectable by conventional NMR due to its
I
DNP-enhanced
17
O (a and b) and
195
Pt
solid-state NMR spectra (c) of surface
species. In (a) a natural abundance
1
H-
17
O
HETCOR spectrum of silica surface
allowed for the distinction of hydrogen-
bonded and non-hydrogen-bonded silanol
sites. In (b) natural-abundance
17
O NMR
enabled, for the first time, the detection of
defect sites in MOFs. In (c)
195
Pt NMR
experiments of surface-bound Pt sites
enabled the unambiguous characterization
of their binding environment.
Higher Speed,
More Precision
B Y B R E E H A N G E R L E M A N L U C C H E S I